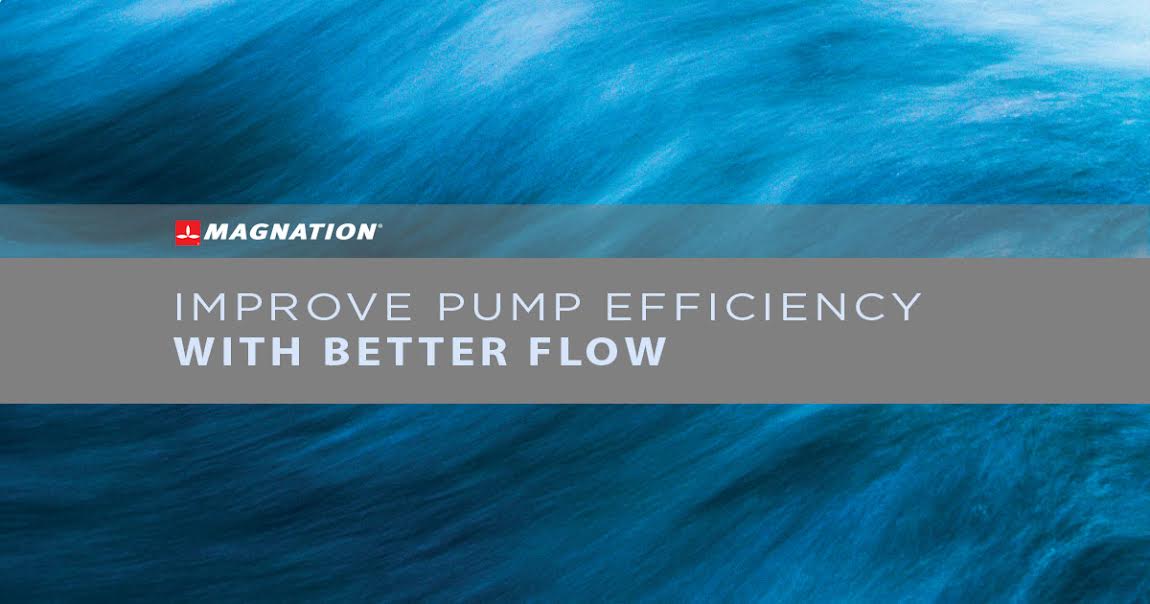
Within the broad category of process pumps, the two types of pumps convert energy through different methods. Positive displacement pumps may use gears, lobes, vanes, air-operated diaphragms, or reciprocating pistons to trap a fluid and then force the fluid through an outlet. The flow rate remains constant.
Centrifugal pumps convert kinetic energy derived from rotating parts to energy and operate in environments that require higher flow rates. Flow and pressure increase through the movement of a vaned impeller rotating within a sealed case.
What Does Energy Efficiency in Pumps Look Like?
In its most basic form, efficiency refers to the ability of the pump to convert rotational or kinetic energy to hydrodynamic energy. The operation of the pump requires mechanical power at the input to produce the desired amount of flow and pressure at the output of the pump. Input power measures the amount of power required to operate the pump. With the energy produced by the pump translating into flow, velocity, and pressure, the output power equals the amount of power actually delivered to the fluid by the pump.
The concept of efficiency describes the ratio between the amount of power found at the output of the pump and the amount of input power found at the pump shaft. A pump that has good-to-excellent efficiency requires less power at the input to produce power at the output. As a result of good efficiency, a pump will have lower operating costs and produce less strain on the motor that turns the pump shaft.
Efficiency Measurements Include Flow, Head, and Pressure
Using a centrifugal pump as an example, the overall efficiency (Ef) equals the ratio of the output or water power (PW) to the input power to turn the shaft that moves the impeller(PS). The ratio appears as:
Ef - Pw / PS
The quantity of PW represents the flow (Q) in gallons per minute multiplied by the head (H) in feet divided by the constant 3960. In equation form, PW appears as:
PW = (Q x H) / 3960
The equation uses the constant to convert the flow multiplied by head to brake horsepower (BHP).
Flow rate describes the rate at which the pump can move fluid through the system. Typically expressed in gallons per minute (gpm), the volumetric flow rate of a system measures the volume of liquid passing a specified point in the system per unit time.
The rated capacity of a pump must match the flow rate required by the application or system. In equation form, the volumetric flow rate shows as the product of the quantity of flow and the average flow velocity or:
Ṽ = Av
with “A” representing the cross-sectional area for flow and “v” representing the average flow velocity. Common units for volumetric flow include gallons per minute, cubic feet per second, cubic centimeters per second, liters per minute, and gallons per hour.
In process pumps, the pressure varies based on the pumped fluid's specific gravity. Because of this factor, most companies and operators use the term “head” to define pump energy. The head equals the column height (feet or meters) above the suction inlet that a pump can lift a fluid. This measurement of system resistance (pressure) remains independent of the fluid's specific gravity. Characteristic curves for pumps often display as head in either feet or meters and convert it to pressure scales such as bar or psi.
Pressure measures the force per unit area of resistance the pump can handle or overcome. Every pump develops a specific pressure in pounds per square inch (PSI) or at a specific flow. The amount of pressure depends on the specific gravity or the weight of the liquid in comparison to water at 20o centigrade. If we divide the head in feet of water by 2.31, we have the pressure in psi. In turn, the pressure in psi multiplied by 2.31 equals the head in feet.
Expressed as the metric unit of pressure “bar,” atmospheres, inches of mercury, millimeters of mercury, torr, or as psi (pounds per square inch), pressure describes influences on fluid behavior. If considered in equation form, pressure equals Force (F) divided by Area (A) or:
Pressure = Force / Area or F/A.
Absolute pressure references the pressure in a vacuum and includes the effects of atmospheric pressure. Examples of absolute pressure measures include altimeters. While gauge pressure references the ambient atmospheric pressure, it excludes the effects of atmospheric pressure. Differential pressure references another pressure point in the system—such as a feed line and a compressor--rather than the ambient pressure.
A pump must have more suction side pressure available than the pump requires for optimal operation. The two parts of Net Positive Suction Head (NPSH)--NPSHA (Available) and NPSHR (Required)--establish the parameters for efficient pump operation. NPSH Available (NPSHA) or the absolute pressure at the suction pump occurs as a function of the system and requires calculation. Pump manufacturers provide the Net Positive Suction Head Required (NPSHR) or the minimum pressure at the suction port of the pump.
The value of NPSHA must always exceed the value of NPSHR to prevent pump cavitation. Calculating the NPSHA requires measurements of the absolute pressure on the liquid in the supply tank (HA), the vertical distance between the lowest liquid level allowed in the tank and the centerline of the pump (HZ), friction losses in the suction piping (HF), and the absolute vapor pressure of the liquid at the pumping temperature (HVP). In equation form, NPSHA appears as:
NPSHA = HA ± HZ – HF - HVP.
Pump Performance Curves Provide Information About Efficiency
All pumps have a performance curve--a graph that illustrates a tremendous amount of information about the pump as it performs under various conditions. In brief, the performance curve shows the pump performance based on head and flow. While the first figure shows a simplified performance curve that shows pump performance based on head and flow, the second figure shows a common example of a manufacturer-provided pump performance curve. The complex set of performance curves indicates possible combinations of total pressure versus volume flow rate on x and y axes.
Simplified Pump Performance Curves
Manufacturer’s Performance Curve
The ratio of energy delivered by the pump to the energy supplied by the pump equals the pump efficiency. When viewing the performance curve shown in the second figure, the efficiency curves intersect with the head-capacity curve. As a result, we know that pump efficiency varies throughout the operating range of the pump. In terms of energy conservation, small improvements in pumping efficiency translate into significant savings of electricity.
Achieving Maximum Efficiency Depends on Eliminating Energy Loss
Process pump efficiency occurs as the product of the mechanical, volumetric, and hydraulic efficiencies. Using centrifugal pumps as an example, the overall efficiency of the pump equals the product of mechanical, volumetric, and hydraulic efficiencies. The capability to maintain each of the three efficiencies depends on the capability to limit energy loss. While mechanical efficiency includes losses that occur through the pump bearing frame, stuffing box and mechanical seals, volumetric efficiency includes losses caused by leakage through the wear rings, balancing holes and--with semi-open impellers--vane clearances. Frictional loss that occurs in the volute of the pump and at the impeller limits hydraulic efficiency.
Every liquid has viscosity--or the resistance to flow. The viscosity--or thickness--of a liquid causes resistance to occur when a fluid moves over the surface of a pipe. Liquids that have higher viscosity have lower flow rates. Water has low viscosity. Yet, several factors can increase the viscosity of water and the resistance to flow. As water becomes colder, viscosity increases. Increasing the pressure of water at higher temperatures also increases viscosity.
Friction loss increases the resistance to flow rate by exerting force in the opposite direction of the flow. As fluid molecules move against each other or against the wall of a pipe, friction loss increases. The drag caused by fluid friction dissipates energy and--in turn--increases costs.
Hard water flowing through any type of piping system carries minerals that deposit on the pipe surface and allow scale to form. Each deposited layer adds friction loss and reduces flow rate. The friction loss introduced by mineral scale also changes the velocity of flowing water and generates turbulence within pipes. While water in the center of a pipe flows at a higher velocity, water close to the rough surface of the pipe flows at a slower rate. To keep water flowing at a higher velocity and to compensate for the difference in velocities while attempting to maintain pressure, systems use more energy.
Magnation Water Technologies Increase Pump Efficiency
Magnation Water Technologies change hard water into soft rain-like water through the application of ionization, magnetism, rifling, vortexing, kinetics, and static mixing. Magnation’s product line improves pump performance by reducing viscosity, therefore energy costs, and produces improved flow and pressure.
Rainbolt (Top) Aquabolt (Bottom)
For more information about Magnation’s products, call (888-) 820-0363 to speak with a water consultant.
Comments
Add Comment